In modern science education, a significant movement encourages students to engage in more than just rote memorization. Rather than focusing solely on static content, teachers and researchers are recognizing the value of fostering scientific thinking in learners. This often involves building science process skills that enable students to investigate, interpret, and critically assess ideas. Many educators see the development of critical thinking as central to scientific exploration, providing a foundation that students can apply not only in higher education but also in everyday life.
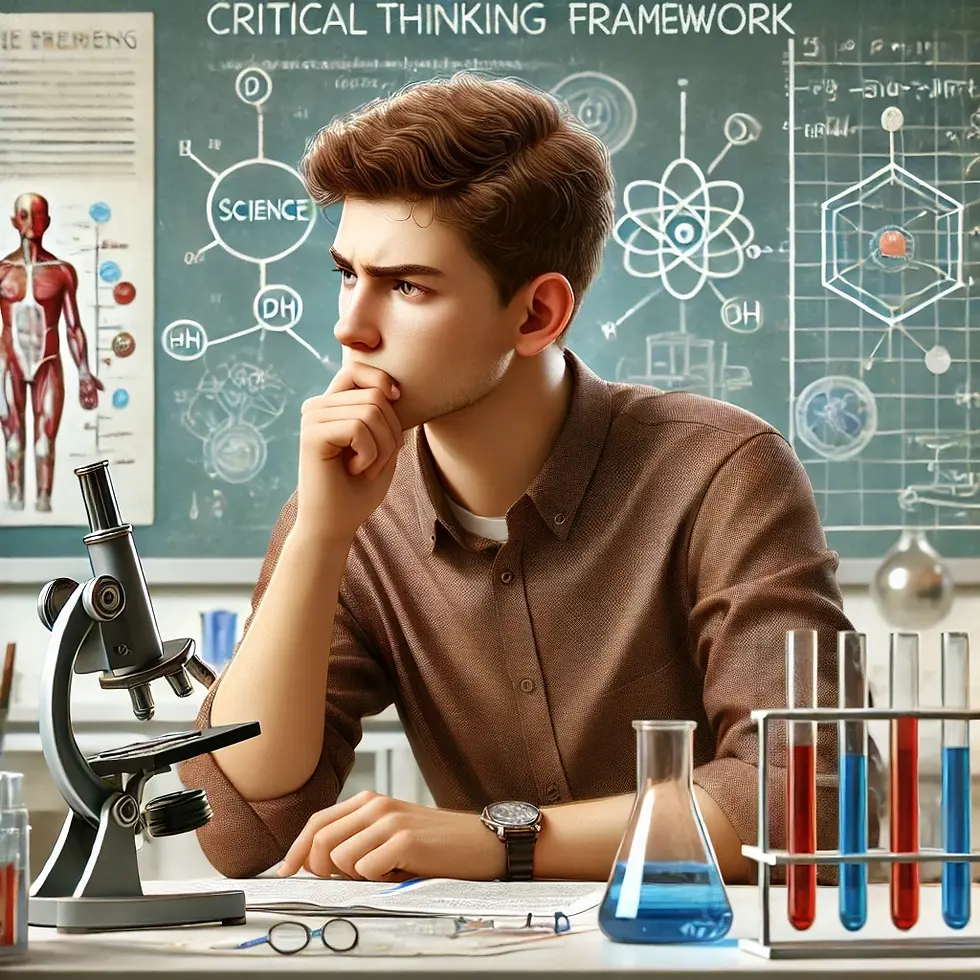
It is within this context that we introduce specialized, science-focused critical thinking frameworks designed for a variety of complex topics. Whether exploring the states of matter, decoding the periodic table, studying the cosmic evolution of massive stars, or dissecting the fundamentals of meiosis & mitosis and bacteria & viruses, these frameworks guide learners through a 9-step process. Each stage is carefully organized into 4 different levels of scaffold, ensuring that students of all ages—ranging from younger students in primary school to senior high school populations—can benefit from structured instruction in scientific reasoning skills.
In recent decades, numerous previous studies in social sciences, cognitive science, and science education have emphasized that embedding critical thinking elements in the learning process can produce a significant difference in students’ outcomes. Through well-researched designs, educators can empower learners to engage more robustly with research questions, weigh empirical evidence, and develop complex reasoning about real world phenomena. This is especially relevant when dealing with challenging scientific concepts such as the lifecycle of massive stars or the structure and function of bacteria & viruses. By building on prior knowledge and introducing new material systematically, students learn to integrate ideas more readily, forming a bridge between what they already know and what they seek to discover.
This blog post thoroughly explores how critical thinking frameworks allow students to become critical thinkers who tackle problem-solving skills in varied contexts, from science courses to social sciences. We will show how these structured frameworks align with established pedagogical models, including the scientific method, theoretical framework analyses, and recognized best practices for science instruction. By referencing insights from the European Journal of Educational Research, the Journal of Technology, the Journal of Physics, and even the Jurnal Pendidikan IPA Indonesia, we weave together a tapestry of current academic thought. From considerations about content knowledge and content validity to reflections on universal intellectual values such as clarity, accuracy, relevance, and depth, this post offers a comprehensive view of why—when students learn using well-developed frameworks—everyone benefits.
Why Critical Thinking Frameworks Matter
To appreciate how students learn using these frameworks, it is essential to consider the impetus behind their creation. Many educators cite the American Philosophical Association in discussing the intellectually disciplined process that underlies robust critical thinking. According to some scholars, critical thinking is about consistently applying intellectual standards—like those derived from the Paul-Elder framework by Richard Paul—to everyday problem-solving scenarios. This approach underscores that critical thinking is not limited to theoretical spaces; it directly informs how students parse fake news, reliable sources, or the multifaceted debates surrounding climate change and global warming.
Furthermore, adopting such frameworks in science education helps cultivate scientific argumentation, which involves marshaling good reasons, evaluating new evidence, and working toward the best solution. In the traditional classroom environment, some might view science as simply learning facts, such as memorizing the periodic table or listing the steps of meiosis & mitosis. However, integrating critical thinking transforms this dynamic. Instead of passively receiving information, students become active investigators, analyzing, synthesizing, and evaluating data drawn from previous studies or empirical study findings.
Critical thinking frameworks also acknowledge the varied cognitive skills involved in learning. Students must develop a capacity for creative thinking, scientific reasoning skills, and communication skills that enable them to articulate their thoughts clearly. Moreover, these frameworks are relevant for high school students, college students, and even pre-service teacher cohorts looking to refine their instructional methods. By presenting research evidence that demonstrates the efficacy of these frameworks, educators are better positioned to advocate for their integration into official lesson plans for science classes.
The Structure of the 9-Step Process
At the heart of these science-focused critical thinking frameworks lies a 9-step process meant to scaffold a learner’s journey toward more sophisticated understanding. While different models might vary in structure, here is an overview that aligns with some commonly accepted phases:
Identify the Big Problem: Students begin by defining or recognizing a major question or concept they want to investigate. This ensures that learning is purposeful and anchored to a clear, motivating issue—akin to forming a research question.
Access Prior Knowledge: Learners reflect on what they already know, bridging content knowledge from previous lessons, everyday life, or even cross-disciplinary experiences. This step acknowledges the power of building on existing cognitive frameworks, as recommended by many a literature review in cognitive science.
Form Hypotheses or Propositions: Students propose potential explanations or solutions. This stage invites creative thinking and highlights that multiple answers could coexist before rigorous testing or examination.
Plan the Investigation: Leaning on science process skills, participants determine how to gather data or explore relevant sources. In many science education contexts, this might include designing an experiment following the scientific procedure or constructing an outline for deeper inquiry.
Collect Data and Empirical Evidence: This step entails actual data-gathering—whether from texts, simulations, or direct experiments. Students often consult reliable sources, peer-reviewed articles, or even observational tasks to accumulate a strong knowledge base.
Analyze and Synthesize Findings: Here, learners practice scientific argumentation by organizing data, scrutinizing results, and connecting them with initial hypotheses. Emphasis on critical evaluation emerges clearly in this phase, tying into the broader ct evaluation framework.
Draw Conclusions: Interpreting results in light of the original big problem, students articulate logical outcomes, refine their perspectives, and potentially revise earlier assumptions. This could involve referencing significant difference found in quantitative data or a conceptual insight gleaned from an exploratory study.
Communicate and Reflect: Sharing findings is crucial. Students at various grade levels practice communication skills by presenting ideas—whether in group discussions, written reports, or digital formats like the journal of technology. Reflection encourages them to see how new insights might apply to other contexts, such as social sciences or the issue of fake news.
Plan Future Inquiry: The final step prompts learners to ask new, deeper research questions, building a cycle of continuous investigation. This fosters a proactive, ongoing approach to learning.
By following this process, students do more than memorize. They engage with intellectually disciplined processes at each phase, reinforcing their ability to function as true critical thinkers.
Four Different Levels of Scaffold
Equally important to the 9-step outline are the 4 different levels of scaffold. These tiers allow learners at various developmental stages, from middle school students to those in higher education, to engage meaningfully with advanced topics like massive stars, bacteria & viruses, or the periodic table. It can also mean that more or less support can be given or longer or shorter inquiry as needed. A typical layering might look like this:
Foundational Inquiry: Students at the earliest levels are guided through the basic definitions and fundamental questions. For topics such as states of matter, this might involve exploring the characteristics of solids, liquids, and gases, alongside simpler tasks like visually identifying examples in everyday environments.
Focused Exploration: As learners progress, they are invited to apply their knowledge to more constrained problem-solving situations. For instance, dealing with meiosis & mitosis might involve analyzing cell division under a microscope or modeling the steps using hands-on activities.
Advanced Analysis and Synthesis: At this level, students interpret data, draw conclusions, and generate new evidence through structured experimentation or deep textual analysis. When looking at massive stars, for example, students might explore how nuclear fusion processes differ from smaller stars or investigate the cosmic implications of supernova events.
Independent Research and Evaluation: The highest stage offers considerable autonomy. Here, learners pose research questions of their own, design investigations, and evaluate results with minimal direct instruction. A key concept might be to discuss climate change or global warming from the vantage point of astrophysics, or to consider how viruses evolve over time in different conditions.
This scaffold ensures that each step of the way, students are exposed to problem-based learning models that develop both lower-order and higher order thinking skills. It also grants teachers and facilitators a clear blueprint for moving learners along a continuum from fundamental comprehension to independent, intellectually disciplined inquiry. Indeed, resources from the journal of physics to the european journal of educational research have long advocated this form of graduated approach to deepen students’ development of critical thinking.
Connecting Frameworks to Real-World Problems
One of the most exciting aspects of these critical thinking frameworks is their adaptability to real world concerns. For instance, when investigating the environmental impacts of climate change, students can practice the 9-step process by formulating hypotheses about rising sea levels or changes in local weather patterns. By grounding the inquiry in empirical study, they gain first-hand experience in verifying scientific claims, cross-referencing global datasets, and distinguishing credible sources from sensationalism—a skill particularly relevant in an age of fake news.
In parallel, exploring global warming through a lens of science instruction can highlight the interplay between content knowledge and social interaction. Students might collaborate as a team (group member roles), assigning tasks like data collection, analysis, or presentation, thus sharpening communication skills. This integrated approach fosters not just academic growth but also social and interpersonal development, aligning with findings about disposition dimensions in critical thinking assessment model research. Ultimately, the learning process becomes richer and more relevant, revealing how scientific inquiry informs public policies, shaping decisions on sustainability, energy usage, or even political debates.
Enabling Problem-Solving Skills in Diverse Science Topics
Science teachers using these frameworks discover that they can apply them effectively across various subject matter divisions. In senior high school chemistry, for example, students might delve into the periodic table, using the 9-step process to unearth relationships between atomic structure, bonding, and chemical reactions. Meanwhile, pre-service chemistry teachers in higher education programs can study how to guide their future classes through a similarly scaffolded approach, ensuring that novices and advanced learners alike develop critical thinking competencies.
Biology teachers can similarly adapt the method for meiosis & mitosis or the study of bacteria & viruses, encouraging learners to see connections between genetic changes, disease processes, and broader ecological cycles. By constantly revisiting steps like hypothesis formation, data collection, and reflection, the frameworks gently nudge students toward seeing themselves as active contributors to scientific conversations. These experiences align with calls in the literature review for deeper immersion in authentic investigative tasks, building both science process skills and an appreciation for the complexity of nature.
Even physics classes can benefit. For instance, investigating the life cycle of massive stars fosters an appreciation not just for cosmic scale but also for how nuclear reactions and astrophysical phenomena tie into advanced mathematics, problem-solving, and engineering. These cross-cutting concepts encourage social interaction as well—students may consult astronomers or reference advanced texts in the Journal of Physics, forging interdisciplinary links that make the lessons memorable and profound.
Building Better Scientific Argumentation and Communication Skills
Central to scientific thinking is the capacity for forming coherent, evidence-based arguments, often referred to as scientific argumentation. In these critical thinking frameworks, every step from identifying a big problem to planning future inquiry offers opportunities to practice persuasive, well-structured communication. Students must justify their claims with good reasons, presenting logically derived conclusions supported by empirical evidence or grounded in established scientific theories. For instance, when analyzing a lab experiment that tests bacterial growth under various pH levels, learners must interpret data, connect it to known microbial behavior, and articulate a conclusion that resonates with recognized theoretical frameworks.
Moreover, as communication skills develop, so does a student’s ability to engage in critical evaluation of other viewpoints. By actively seeking out reliable sources, learners discover how data can be manipulated or misrepresented, a vital skill in combating the spread of fake news. Students become adept at questioning assumptions and biases—both in themselves and in others—ultimately leading them to a more balanced understanding of real world phenomena, from medical breakthroughs to planetary challenges like global warming.
The Role of Previous Studies and Empirical Evidence
No robust discussion of critical thinking frameworks can ignore the weight of previous studies, research study findings, and other forms of academic inquiry. According to many experts in science education, the development of students is optimized when teaching strategies draw on validated methods. This is particularly important for educators designing science courses or programs for senior high school learners and college students. By examining studies in the European Journal of Educational Research, educators can learn how systematically structured interventions, such as problem-based learning and scaffolded inquiry, yield beneficial effects on both science achievement and overall critical thinking test performance.
Empirical study also underlies the best practices for ct skills cultivation. For example, the california critical thinking skills test is a commonly used instrument to measure how well students can interpret, analyze, and evaluate various forms of information. When teachers integrate frameworks that systematically move students through each step of reasoning, these test scores often reflect a tangible rise in critical thinking proficiency. Another recognized tool is the critical thinking assessment model, which can be customized to different contexts—whether for middle school science, pre-service chemistry teachers, or advanced seminars in political science.
These frameworks likewise resonate with the concept of an integrated critical thinking framework that has gained traction in the American Philosophical Association and M. A. or M. J. theses. The main rationale is that bridging multiple theories—such as the Paul-Elder framework, Richard Paul’s philosophies, and other established approaches—can amplify the transformative impact on student learning. The synergy between structured inquiry steps, scaffolded knowledge levels, and evidence-based pedagogical methods underscores the overarching mission of modern science education: to create not merely content-receptive students but genuinely curious, analytical individuals.
Cultivating Disposition Dimensions and Intellectually Disciplined Process
Aside from cognitive skills, an equally important facet of learning involves disposition, meaning the attitudes, motivations, and willingness students bring to the table. Disposition dimensions such as open-mindedness, skepticism, and perseverance often distinguish truly critical thinkers from those who can replicate steps superficially but lack deeper engagement. Critical thinking frameworks that use incremental steps ensure repeated practice, reinforcing these dispositions over time. Indeed, when students confront big scientific problems—like how to mitigate global warming—they not only apply what they know but also cultivate habits of mind that embrace complexity and nuance.
In this context, the term intellectually disciplined process becomes more than a buzzword. It signals a dedicated, structured effort to maintain rigor at every stage of inquiry. Students learn that guessing or skipping steps undermines the validity of their conclusions, whereas a methodical approach fosters robust findings. Over time, these frameworks help shape an internal sense of responsibility for accuracy and clarity—hallmarks of universal intellectual values. Learners can then transfer these attitudes to other domains, from mathematics to social sciences, and even across the boundary of academia into professional life.
A Closer Look at Science-Focused Topics
To illustrate how these frameworks operate in practice, let’s delve more deeply into a few science topics:
States of Matter: Often taught in primary school or early middle school, the concept of solids, liquids, and gases introduces students to fundamental properties that shape all physical phenomena. Using the 9-step process, learners might hypothesize about how heat energy affects molecular movement. They gather empirical evidence via simple experiments—like monitoring water transitioning from liquid to gas—and develop an argument based on observed data.
Periodic Table: In high school students or senior high school contexts, the periodic table can be explored through a lens of elemental properties, electron configurations, and chemical bonding. Through the framework’s scaffolded inquiry, learners compare elements, question periodic trends, and design lab investigations that test real chemical reactions. Each step fosters deeper comprehension of how the table is organized, bridging prior knowledge with new evidence gleaned from experiments or literature.
Massive Stars: Astronomy topics push the boundaries of what many students conceive as everyday science. Investigating the life cycle of massive stars—how they fuse elements, expand, and eventually might explode as supernovae—can inspire a sense of wonder and highlight interdisciplinary connections. The frameworks encourage learners to propose explanations for gravitational collapse or to model the effect of fusion rates. This merges science instruction with imaginative leaps, reinforcing how creative thinking and critical thinking intersect.
Meiosis & Mitosis: These crucial biological processes are often taught in middle school or high school biology classes. Students use the 9-step method to identify the significance of cell division, gather data from microscope slides, and interpret how chromosomal crossovers affect genetics. This fosters a robust understanding that transcends memorizing phases—learners see the broader context and implications, preparing them for advanced genetics courses later in their academic journey.
Bacteria & Viruses: Especially relevant in discussions around global health, disease outbreaks, and broader ecosystem functionality, the study of bacteria and viruses can be infused with problem-based learning. Students might examine how viruses replicate, how bacterial communities respond to antibiotics, or the role of microorganisms in climate patterns. Each step of the framework reminds learners to check for reliable sources, evaluate the data they gather, and articulate their conclusions carefully, thereby building the foundation for scientifically literate citizens.
In each case, learners discover that an important step is not just to get the right answer, but to walk through a rigorous inquiry—posing a hypothesis, testing it, and reflecting critically on results. This orientation trains them to become active participants in constructing scientific knowledge rather than mere observers.
The Role of Social Interaction and Group Work
Although critical thinking is often associated with individual mental activity, many frameworks place a premium on social interaction. When students collaborate, they inevitably bring different perspectives to the table, challenging each other’s assumptions and refining each other’s reasoning. Through structured group dialogues, participants share evidence, debate interpretations, and co-construct knowledge. This communal approach also helps address the disposition dimensions—learners practice collegiality, openness, and empathy, recognizing that science is rarely an isolated pursuit.
Moreover, diverse teams provide a richer environment for diagnosing misconceptions, brainstorming creative solutions, and ensuring a more robust final product. Such an approach mirrors what professionals do in scientific fields—laboratories worldwide rely on collaborative teams where each group member contributes expertise. Facilitators can harness this dynamic by distributing tasks aligned with the steps of the 9-step process or the problem-based learning model, ensuring each student plays a part in the investigative journey.
Linking Back to Research Study, Empirical Study, and Literature Review
One of the strongest endorsements for using structured frameworks arises from a solid body of research study and literature review. Investigations published in the European Journal of Educational Research and the Jurnal Pendidikan IPA Indonesia consistently highlight that methodical approaches to science learning can yield better outcomes. By weaving critical thinking into subject matter divisions like physics, chemistry, biology, or even social sciences, educators observe improvements in content mastery, critical thinking test scores, and overall engagement.
Studies focusing on pre-service teacher populations underline these benefits, too. When teachers-in-training learn how to implement scaffolded frameworks, they are more likely to produce well-structured lesson plans that incorporate each phase of inquiry—leading to better science achievement among their future students. The paul-elder framework, along with strategies informed by richard paul, provide robust theoretical foundations that can be adapted to various classroom contexts. Meanwhile, references to the journal of physics or the journal of technology show how solutions can be technologically integrated—like using online labs, simulations, or data analysis tools—to enrich the learning process.
The Role of Problem-Based Learning and CT Evaluation Frameworks
An evolution of these ideas appears in the problem-based learning model. This approach situates students in authentic or simulated real-world issues. For instance, in a scenario regarding global warming, students might assume the roles of climate scientists, city planners, or environmental activists. They then move through the 9-step process—defining the question, reviewing empirical evidence, forming potential solutions, and ultimately discussing how outcomes could be implemented. Such tasks weave in seamlessly with ct evaluation frameworks, where each step or milestone is assessed for clarity, depth, breadth, and the other universal intellectual values.
Furthermore, the integration of social sciences highlights how problems rarely exist in a vacuum. A city grappling with rising temperatures must consider community well-being, economics, historical factors, and political implications. This leads to an intersection of political science and science education, where students learn that critical thinking is an interdisciplinary skill set. It acknowledges the multi-faceted nature of real-world problems and encourages future generations to adopt a holistic perspective.
Making It Concrete with Assessments
Naturally, teachers and administrators may ask how to measure whether these frameworks genuinely deepen critical thinking skills. Here, tools like the california critical thinking skills test offer a standardized lens. Pairing the test results with a thorough analysis of student development in group discussions, lab reports, or culminating projects can indicate whether the framework is fostering improved reasoning. Another factor is the alignment of assignments with content validity standards to ensure that the tasks indeed measure relevant scientific concepts alongside thinking dispositions.
Educational researchers might conduct their own empirical study to track how cohorts progress after exposure to such frameworks versus traditional lecture-based methods. They could also examine whether improvements persist over time, shedding light on the longer-term impact of scaffolded critical thinking. Indeed, m. a. or m. j. level theses frequently delve into precisely these types of questions, investigating the interplay between teaching methodology and learning process outcomes. Over the years, comprehensive literature reviews have found that systematically embedding critical thinking fosters more profound scientific comprehension, deeper student engagement, and heightened capacity for evaluating fake news or unsubstantiated claims.
Addressing Younger Students and Primary School Contexts
Although many references in this post focus on high school students, senior high school, or college students, the frameworks can be introduced at much earlier stages as well. Younger students in primary school may begin learning about states of matter or basic life processes through simplified versions of the 9-step inquiry. They benefit enormously from seeing that science is an active, questioning discipline. Even if their content knowledge is minimal at this stage, the habit of systematically investigating the world can spark lifelong curiosity.
Teachers who integrate small-scale experiments or guided inquiry sessions—like exploring how water freezes or boils—instill the principle that answers come from data and reasoning, not merely from authority figures or guesswork. Over time, these students enter middle school and then high school with an ingrained sense that science is iterative and that each new question invites further exploration. Their minds become accustomed to an intellectually disciplined process, setting the stage for advanced topics later on.
Potential Challenges and Common Misconceptions
Implementing systematic critical thinking frameworks is not without challenges. Some educators may worry that they lack the training or time to design multi-step inquiries across multiple subject matter divisions. Others may fear that students who prioritize quick answers might resist the reflective depth required by each stage. Indeed, many a research study notes that students used to passive learning initially struggle when asked to formulate hypotheses or evaluate contradictory evidence.
However, these obstacles often give way to success once learners experience the satisfaction of deeper understanding. Through consistent practice, they realize the inherent power of an evidence-based approach. Another misconception is that critical thinking only applies to advanced or gifted students. On the contrary, younger or middle school students can handle simplified frameworks if the scaffolding is appropriate. Even the fundamental act of noticing patterns, questioning results, and verifying with observation fosters the seeds of critical thinking.
The Future of Critical Thinking in Science Education
Looking ahead, many proponents argue that the ultimate goal of modern science education should be a generation of students equipped with robust, flexible critical thinking capabilities. These learners, whether in higher education or just entering the workforce, carry within them an awareness that problems must be understood from various angles and tested against a tapestry of empirical evidence. They also recognize that knowledge is always evolving—today’s facts might be refined tomorrow by new evidence.
In a rapidly changing world where fake news proliferates online and critical decisions must be made about topics like climate change, global warming, or public health, the importance of a scientifically literate and thoughtful populace cannot be overstated. A focus on good reasons, reliable sources, and practical applications of knowledge fortifies societies against misinformation and fosters meaningful innovation.
At the same time, these frameworks reflect the best impulses of the scientific method, which demands that claims be testable, data be interpretable, and all conclusions remain open to revision when confronted with contradictory evidence. This spirit of inquiry stands at the heart of what it means to be a critical thinker in both everyday life and professional spheres. It is encouraging to note the rising popularity of such structured approaches in teacher education, the journal of technology, and science instruction conferences globally.
Conclusion
Ultimately, how students learn using critical thinking frameworks comes down to a synergy between structured inquiry, subject matter divisions, careful scaffolding, and ongoing assessment of both cognitive and dispositional growth. By engaging in a 9-step process that begins with defining big questions and ends with planning future inquiries, students learn that science is neither static nor purely fact-based. Instead, it is a dynamic interplay of hypothesis, evidence, debate, and discovery.
The 4 different levels of scaffold enable each learner—from primary school novices tackling states of matter to senior high school explorers investigating massive stars—to find a comfortable yet challenging entry point. Whether they are confronting complexities of bacteria & viruses or analyzing the intricacies of meiosis & mitosis, this approach ensures thorough grounding in scientific concepts, methods, and reasoning. Coupled with guidance from teachers familiar with universal intellectual values and mindful of ct evaluation framework principles, students become increasingly confident in their ability to connect theory with real world problems.
Looking at previous studies in the European Journal of Educational Research, the Journal of Physics, and the Jurnal Pendidikan IPA Indonesia, it is clear that systematic instruction focusing on scientific argumentation, communication skills, and critical scrutiny yields a significant difference in students’ development. Over time, these learners exhibit greater independence in constructing knowledge, a stronger capacity for critical evaluation, and more resilient problem-solving skills. Indeed, the entire learning process is invigorated when content mastery is intimately tied to robust cognitive and affective engagement.
Such achievements are not limited to science alone; they spill over into social sciences, mathematics, political science, and beyond. After all, cognitive processes underlie our interactions with the world, shaping how we interpret news, interact with peers, and address ethical dilemmas. The adoption of critical thinking frameworks can lead to individuals who are not only academically successful but also socially responsible and creatively engaged with the pressing challenges of our time—be it verifying the authenticity of a study on global warming or constructing a thoughtful discussion around genetics and ethics.
From middle school students excitedly exploring microworlds to college students designing sophisticated experiments, the consistent theme is one of intellectual growth. Learners discover that the ability to harness scientific reasoning skills is not an end in itself, but a gateway to continuous inquiry and reflection. The same is true for pre-service teacher cohorts, who pass along these methods to the next generation, ensuring that each wave of students is better prepared than the last to question assumptions, weigh evidence, and shape the world around them.
In conclusion, science education that incorporates well-structured, 9-step critical thinking processes offers a pathway toward nurturing critical thinkers who flourish in everyday life, higher education, and beyond. It merges theory and practice in a way that highlights good reasons for adopting the frameworks, from increased retention and engagement to the cultivation of an intellectually disciplined process. As more science teachers embrace these strategies, we can expect to see a broadening impact on students’ development, science learning outcomes, and the overall purpose of this study in shaping a more literate and thoughtful society.
By applying these frameworks—whether investigating massive stars, dissecting the function of bacteria & viruses, or analyzing states of matter—students consistently refine their thinking patterns. The evidence points strongly to their efficacy in improving critical thinking measures, encouraging self-directed exploration, and setting learners on a path toward lifelong curiosity. Indeed, as the educational landscape continues to evolve in tandem with technological and scientific advances, fostering robust critical thinking among future generations stands as an indispensable goal, ensuring that students can navigate not only the frontiers of science but the complexities of an ever-changing world.
Thanks for reading
Cheers and stay curious
Oliver - The Teaching Astrophysicist
Comments